UCSD Biologists Visualize Protein Gradient Responsible for Dividing Embryo into Nervous System, Epidermis
January 15, 2002
By: Kim McDonald
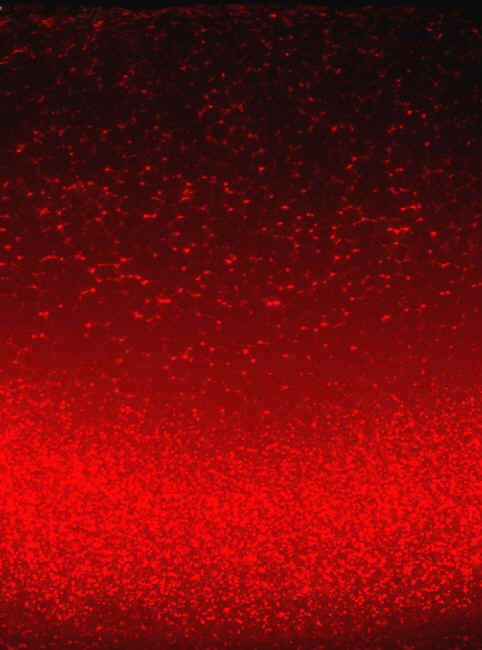
Graphic showing division of dorsal region of developing embryo (yellow) caused by protein gradient (color photo)
Graphic Credit: Ethan Bier. Photo Credit: Shaila Srinivasan
Biologists at the University of California, San Diego have observed, for the first time, a protein gradient in developing fruit fly embryos believed to trigger the division of the embryo into nervous system and different types of epidermis within complex organisms like humans.
In a paper featured on the cover of this month's issue of the journal Developmental Cell, the scientists demonstrate visually and in experimental detail the molecular process by which an embryo begins partitioning itself for subsequent development into neural and distinct forms of epidermal tissue. Their experiments provide final confirmation of an elegant hypothesis proposed during the 1950s by the mathematician Alan Turing, who suggested that chemicals generated incrementally during the development of a complex organism might cause the differentiation of cells during early embryonic development.
"We are now one step closer to understanding the mechanism by which crude spatial information provided by the egg is converted into more refined information that ultimately defines every position along the body axis in exquisite detail," says Ethan Bier, a professor of biology at UCSD who headed the research. "This process assures that fingernails grow only on the tips of fingers and two eyes become positioned symmetrically on either side of the nose."
Turing suggested that the chemicals responsible for the developmental changes in the embryo, which he dubbed "morphogens," were produced and secreted by one group of cells, then diffused into neighboring cells where they were destroyed a certain rate. According to Turing, the juxtaposition of cells producing the morphogen with adjacent cells acting as a sink to remove that substance would create a balanced situation leading varying concentrations of that substance depending on its distance from the source. Cells close to the source would be exposed to high levels of the morphogen while those further away would see lower levels. Those differences, Turing thought, would provide the instructions to differentiate cells during development.
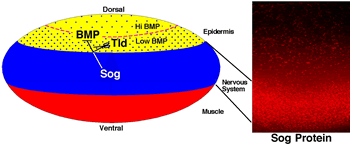
Turing's idea spurred a search for these morphogens and, over the past two decades, several proteins were identified that appear to possess many of the characteristics he proposed for morphogens. One these proteins, which is known to promote the early development of the nervous system in animals as diverse as humans and insects, is called Chordin in humans and Short Gastrulation, or Sog for short, in fruit flies.
Chordin and Sog, sometimes referred to as neural inducers, work by binding to and inactivating the effect of other secreted factors known as BMPs. The neural inducers prevent BMPs from actively suppressing the default preference of the embryonic cells, which is to become neural tissue.
"Neural inducers have remarkably similar functions in insects and humans as highlighted by the fact that one can inject Sog into the region of a frog embryo that normally would give rise to epidermis and induce the formation of a second frog nervous system at that position," says Bier.
Neural inducers also have an effect on the kind of epidermal tissue, which forms next to the nervous system. Consistent with Turing's model, some scientists believed that Sog diffused into neighboring epidermal cells to suppress BMP activity, creating two zones of differential BMP activity—one of which becomes epidermis proper and the other of which forms a tissue similar to amniotic tissue. However, no one was able to demonstrate that Sog was present in a graded fashion as predicted by this model.
The reason, it turned out, was that the levels of diffusing Sog protein were much too low to be detected by available histochemical methods. Shaila Srinivasan, a postdoctoral researcher in Bier's laboratory, overcame that impediment by developing a sensitive fluorescence detection technique that made it possible to directly observe the accumulation of Sog outside of the area in which it is synthesized.
"Having established that we could visualize the graded distribution of Sog in normal embryos, we then tested the hypothesis that an enzyme produced in the epidermal region called Tolloid, which cleaves and inactivates Sog, might be responsible for this," says Bier, whose work was financed by the National Institutes of Health. "We did this by examining the pattern of Sog accumulation in mutants lacking function of the Tolloid gene. We observed that in the absence of Tolloid, Sog levels are greatly elevated in epidermal cells and accumulate steadily until the entire embryo contains nearly uniform amounts of Sog. We also found another cellular function that limits epidermal Sog levels—a process known as endocytosis, through which proteins are taken up from the outside of the cell. These studies provide the final link demonstrating that Sog is a morphogen and explain how the graded distribution of Sog is created."