Samara Reck-Peterson
Research
The contents of eukaryotic cells are highly dynamic yet organized spatially and temporally. This is achieved primarily by the microtubule cytoskeleton and associated transport machinery, whose fundamental nature is highlighted by the many neurological diseases caused by mutations in them. The overarching goal of the Reck-Peterson lab is to understand how this system works at molecular, cellular, and organismal scales.
The Reck-Peterson lab is highly interdisciplinary, and we use in vitro biochemical reconstitution, protein engineering, single-molecule imaging, proteomics, genoomics, live-cell imaging, and genetics to achieve our goals. Through collaborative projects with others at UC San Diego we use cryo-electron microscopy (with Andres Leschziner’s lab) and cryo-electron tomography (with Elizabeth Villa’s lab) to incorporate a structure-guided approach to understanding intracellular transport, and we develop testable quantitative physical models of transport (with Elena Koslover’s lab).
The Reck-Peterson lab has made major contributions to determining how the dynein motor works and is regulated, to developing tools and screening strategies to study bi-directional movement of cargos on microtubules, to understanding the regulation of intracellular transport in cells, to determine how defects in microtubule-based trafficking may impact Parkinson’s Disease. Current research directions in the lab include:
(1) How does the dynein motor work? Dynein is a large and complicated molecular machine. We have made contributions to developing recombinant systems to express dynein, determining how dynein steps along microtubules, and determining how it is regulated by Lis1. Both the dynein motor encoding gene (DYNC1H1) and the LIS1 gene are mutated in the neurodevelopmental disease lissencephaly. We have shown that the Lis1 protein binds directly to dynein’s motor domain and impacts its mechanochemical cycle (dynein is a AAA ATPase and its nucleotide cycle controls its interactions with its microtubule track). We also discovered that Lis1 promotes the formation of activated dynein complexes. Activated dynein complexes include 12 dynein subunits, 23 dynactin subunits and a dimeric “activating adaptor”. Current efforts in the lab are focused on determining how Lis1 promotes the formation of these massive, activated dynein complexes. For example, in our most recent work in collaboration with the Leschziner lab we captured a structural intermediate in the Lis1-mediated activation of dynein.
(2) What is the mechanism and function of organelle hitchhiking? We co-discovered the phenomenon of organelle hitchhiking. Using a genetic screen designed to determine how peroxisomes link to molecular motors for movement, we unexpectedly discovered that peroxisomes do not directly recruit dynein and kinesin, but rather hitchhike on motile endosomes. We identified a putative molecular tether (PxdA) and a phosphatase (DipA) that are required for peroxisome hitchhiking. Current efforts are focused on determine how and why peroxisomes hitchhike on early endosomes.
(3) Why do perturbations to the transport machinery cause disease? Mutations in many components of the transport machinery (the motors, the tracks and regulators of both) are directly linked to a wide variety of neurodevelopmental and neurodegenerative diseases. In addition to our studies on Lis1, we recently began working on LRRK2. The LRRK2 gene is one of the most commonly mutated genes in familial Parkinson’s disease. In collaboration with the Leschziner lab, we showed that LRRK2 binds directly to microtubules in the absence of any other proteins. By doing so it can act as a “roadblock” for dynein and kinesin microtubule-based motors, nearly completely abolishing their movement at low nanomolar concentrations. LRRK2 binds to microtubules at lower concentrations when it carries Parkinson’s Disease mutations or in the presence of LRRK2-specific Type 1 kinase inhibitors. Because Type 1 kinase inhibitors are being investigated for the treatment of Parkinson’s Disease, current efforts in the lab are aimed at determining if endogenous LRRK2 interacts with microtubules in the presence of kinase inhibitors.
(4) RNA recoding of molecular motors in cephalopods: Cephalopods such as squid and octopus extensively use Adenosine to Inosine RNA editing to edit their messenger RNAs, which can result in recoding (amino acid changes at the protein level). The transport machinery that we study is extensively recoded. We discovered that this recoding increases in the cold by studying recoding in wild squid. Further, we found that kinesin motors carrying recoded sites were “better” motors in the cold- they were more likely to interact with microtubules when carrying cold-specific recoded sites. We also showed that sites of recoding may serve as a roadmap for identifying regions of proteins that can be functionally modified, suggesting that the cephalopod “editomes” could serve as a guide for understanding the function of proteins that are conserved between cephalopods and other organisms. Currently, we are developing a database to explore how the squid transcriptome changes in response to temperature based on experiments with wild squid.
(5) Other directions: We are always exploring new directions related to transport in a variety of organisms using a wide range of techniques and methods. If you are interested in joining our team, contact Sam to discuss your project ideas.
Go to full publication list
Select Publications
- Rangan KJ‡ and Reck-Peterson, SL‡. (2023) RNA recoding in cephalopods tailors microtubule motor protein function. Cell 186: 2531-2543.
- Highlighted by Koenig, KM. (2023) Chilling with cephalopods: Temperature-responsive RNA editing in octopus and squid. Cell 186: 2518-2520.
- Snead DM*, Matyszewski M*, Dickey AM*, Lin YX, Leschziner AL‡, Reck-Peterson SL‡. (2022) Structural basis for Parkinson’s Disease-linked LRRK2’s binding to microtubules. Nat Struct Mol Biol. 12: 1196-1207.
- Stevens DA*, Beierschmitt C*, Mahesula S, Corley MR, Salogiannis J, Tsu BV, Cao B, Ryan AP, Hakozawki H, Reck-Peterson SL‡, and Daugherty MD‡. (2022) Antiviral function and viral antagonism of the rapidly evolving dynein activating adaptor NINL. eLife 11: e81606.
- Deniston CK*, Salogiannis J*, Mathea S*, Snead DM, Lahiri I, Matyszewski M, Donosa O, Watanabe R, Bohning J, Shiau AK, Knapp S, Villa E, Reck-Peterson SL‡, Leschziner AL‡. (2020) Parkinson’s Disease-linked LRRK2 structure and model for microtubule interaction. Nature 588: 344-349.
- Hot Topics: Olszewska and Lang (2020). “Opening” new insights into LRRK2 conformation and the microtubule”. Movement Disorders 35: 2162-2163.
- Htet ZM*, Gillies JP*, Baker RW, Leschziner AL, DeSantis ME‡, Reck-Peterson SL‡. (2020) Lis1 promotes the formation of maximally activated cytoplasmic dynein-1 complexes. Nature Cell Biology 5: 518-525.
- News and Views: McKenney RJ (2020). Lis1 cracks open dynein. Nat Cell Biol. 22: 515-517.
- Reck-Peterson SL‡, Redwine WB, Vale RD, Carter AP‡. (2018) The cytoplasmic dynein transport machinery and its many cargoes. Nat Rev Mol Cell Biol. doi: 10.1038/s41580-018-0004-3.
- Redwine WB*, DeSantis ME*, Hollyer I, Htet ZM, Tran PT, Swanson SK, Florens L, Washburn MP, Reck-Peterson SL. (2017) The human cytoplasmic dynein interactome reveals novel activators of motility. eLife 6:e28257.
- Salogiannis J, Egan MJ, Reck-Peterson SL. (2016) Peroxisomes move by hitchhiking on early endosomes using the novel linker protein PxdA. J Cell Biol 212: 289-296.
- In this issue: Short B. (2016) PxdA helps peroxisomes hitch a ride. J Cell Biol 212: 258.
- Research highlight: Strzyz P. (2016) How peroxisomes hitchhike on endosomes. Nature Reviews Mol Cell Biol. 17: 134.
- Derr ND*, Goodman BS*, Jungmann R, Leschziner AE, Shih WM, Reck-Peterson SL. (2012) Tug of-war in motor protein ensembles revealed with a programmable DNA origami scaffold. Science 338: 662-665.
- Perspective: Diehl MR. (2012). Templating a Molecular Tug-of-War. Science 338: 626-627.
- Huang J*, Robert A*, Leschziner L, Reck-Peterson SL. (2012) Lis1 acts as a "clutch" between the ATPase and microtubule-binding domains of the dynein motor. Cell 150: 975-986.
- Preview: Trokter M and Surrey T. (2012) LIS1 Clamps Dynein to the Microtubule. Cell 150: 877- 879.
* | These authors contributed equally |
---|---|
‡ | Co-corresponding authors |
Bold | Reck-Peterson lab members |
Biography
Dr. Sam Reck-Peterson received her B.A. degree in Biology at Carleton College, her Ph.D. in Cell Biology at Yale University and did postdoctoral research at the University of California San Francisco. She joined the faculty at Harvard Medical School in 2007, where she was an Assistant and then Associate professor of Cell Biology. She was recruited to UC San Diego in 2015. Dr. Reck-Peterson was the recipient of an NIH New Innovator Award (2008), a Rita Allen and Milton Cassel Scholar award (2009), and a Howard Hughes Medical Institute Simons Faculty Scholar Award (2016). She became an Investigator of the Howard Hughes Medical Institute in 2018. In addition to HHMI, her research is funded by the National Institutes of Health, and Aligning Science Across Parkinson’s. She received the American Society for Cell Biology (ASCB) WICB Junior Award for Excellence in Research in 2013 and was named an ASCB Fellow in 2023.
Dr. Reck-Peterson is a mechanistic cell biologist. Her research focuses on the molecular basis of intracellular transport along cellular tracks called microtubules. Her work has revealed how the microtubule-based molecular motor “dynein” works and is regulated. She also studies how defects in intracellular transport are linked to disease. For example, her team is investigating the links between Parkinson’s Disease and microtubule-based transport, as well as how viruses subvert the dynein machinery to escape immune responses. Dr. Reck-Peterson served as a council member for the American Society for Cell Biology (ASCB) from 2014 to 2017, was the Chair of the 2018 Gordon Research Conference on Cytoskeletal Motors and the Program Chair for the 2018 ASCB|European Molecular Biology Organization (EMBO) joint annual meeting. She serves on the editorial boards of the Journal of Cell Biology and Annual Reviews in Cell and Developmental Biology.
Dr. Reck-Peterson is passionate about making cutting-edge technology available to her local scientific community. She was involved in establishing the Nikon Imaging Center at UC San Diego in 2018 and serves as its Faculty Director. She was also involved in establishing the Goeddel Family Technology Sandbox in 2022 and served as its co-Director until 2023. Together, these centers bring advanced light microscopy, cryo-electron microscopy, cryo-electron tomography, mass spectrometry and flow cytometry resources to the UC San Diego community.
Dr. Reck-Peterson is also passionate about training and mentoring the next generation of scientists. She was the Associate Director of the Biological and Biomedical Sciences Graduate Program at Harvard Medical School from 2011 to 2014. She received the “Young Mentor Award” from Harvard Medical School in 2012. She reviews grants for the HHMI Hannah Gray Fellows Program, the HHMI Freeman Hrabowski Program, and is on the Board of Scientific Advisors for the Jane Coffin Childs Postdoctoral Fellowship—all programs that support early career scientists.
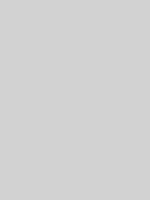