Xin Sun
Research
The building of an organ starts from an amorphous cluster of cells, and proceeds following an elaborate blueprint of coordinated proliferation, morphogenesis and differentiation. Organ function relies on harmonization with other tissues, the infusion of immune cells, and the wiring by the nervous system. We employ cutting-edge technologies, including single-cell transcriptomics and epigenomics, genome editing, whole-organ clearing and imaging, to delineate the robustness that dictates normal organ function, and uncover the imperfections that initiate pathogenesis.
At the center of our investigation is the lung, an organ that is vital at first breath. It is the setting for many fetal, pediatric and adult diseases with unknown cause and no cure. We use disease modeling as a starting point for fundamental discoveries in developmental biology, stem cell biology and the neural-immune-physiology interface.
Consider the Lung As a Sensory Organ
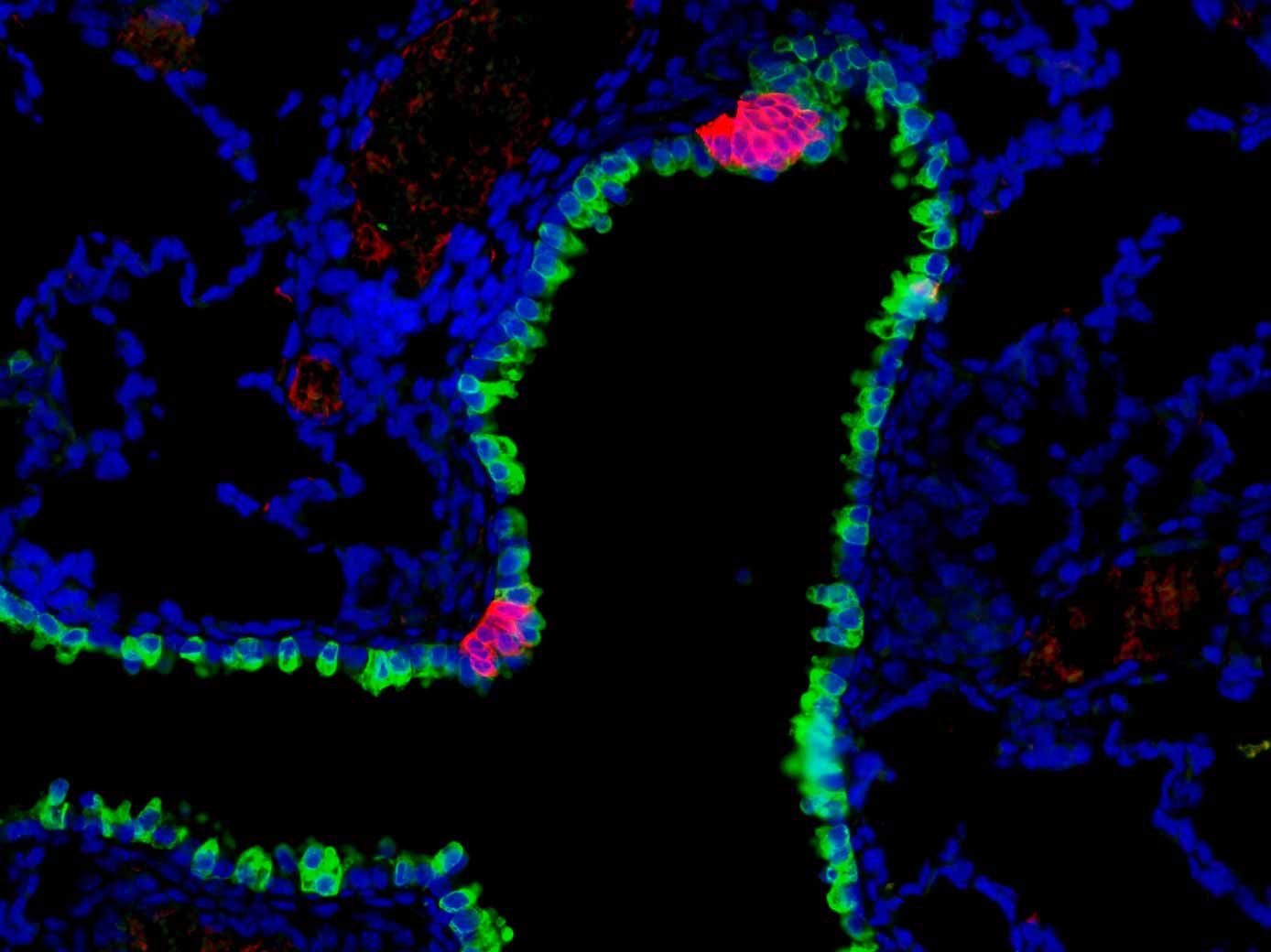
A mouse airway outlined by an epithelial marker (green), annotated by clusters of pulmonary neuroendocrine cells (magenta) which act as airway sensors.
We study Interoception, the process of sensing self. We focus on the lung, an organ that is vital for survival at first breath, and a frequent target of infection including COVID19. At resting, an average person inhales/exhales 5-8 litters of air. This air may vary in oxygen content, carry allergen, pollutants or pathogens. Each of these signals would trigger a specific set of response. We study how the specificity is achieved through intricate organ, neural and immune interactions.
We found that pulmonary neuroendocrine cells, a rare cell type in lung, function as sensors on the airway wall (Branchfield et al., Science, 2016) . These cells can be activated by signals such as allergen, which in turn secrete potent neuropeptides and neurotransmitters to trigger immune responses (Sui et al., Science, 2018) . Through modeling a rare disease with increased neuroendocrine cells, we found that excess neuropeptides from these cells can cause vessel leakage in lung, obstructing gas exchange (Xu et al., Developmental Cell, 2022) . Study of the pulmonary neuroendocrine cells and their innervation by nerves also led us to map the neural circuits that control lung function (Su, Barr et al., AJP Lung, 2022) .
Deciphering Human Diseases at Single Cell Resolution
As members of the NHLBI LungMAP consortium, our team generated the first open access normal human lung donor-matched single nucleus RNAseq and single nucleus ATACseq datasets (LungMAP.net, Wang et al., eLife, 2020, Sun et al., Developmental Cell, 2021) . These datasets serve as blueprint for the generation of single cell atlas of diseased lungs, with our focus on pediatric lung diseases.
We also use CRISPR/Cas9 genome editing to generate mouse models carrying patient mutations, and use them to study lung disease mechanisms. We have studied a number of lung-related congenital disorders, including tracheo-esophageal fistula (Domyan et al., Development 2011) , tracheobronchomalacia (Hines et al., PNAS 2013) , and congenital diaphragmatic hernia (CDH) (Domyan et al., Dev Cell, 2013; Branchfield et al., Science, 2016, McCulley et al., JCI, 2018) . Elucidation of disease mechanisms also led to novel basic biology discoveries.
Stem Cells in Development and Injury Repair
We study fundamental questions pertaining to organ stem cells, in how they form in development, how they drive tissue repair, and how they can become dysplastic and initiate disease (Nantie et al., Development, 2018; Li et al., Developmental Biology, 2021) . We also study how stem cells interact with surrounding niche cells to construct airway and gas exchange units (Branchfield et al., Dev Biol, 2015; Li et al., eLife, 2018; Li et al., JCI, 2020; Young et al., Developmental Cell, 2020) . Disruption of these processes leads to alveolar simplification, as is seen in premature infants diagnosed with bronchopulmonary dysplasia, a condition often associated with lifelong breathing deficiencies, pulmonary hypertension and accelerated decline in respiratory capacity.
Select Publications
-
- Xu J, Xu L, Sui P, Chen J, Moya EA, Hume P, Janssen WJ, Duran JM, Thistlethwaite P, Carlin A, Gulleman P, Banaschewski B, Goldy MK, Yuan JX, Malhotra A, Pryhuber G, Crotty-Alexander L, Deutsch G, Young LR, Sun X. Excess neuropeptides in lung signal through endothelial cells to impair gas exchange. Dev Cell. 2022 Apr 11;57(7):839-853.e6. Featured Article. doi:10.1016/j.devcel.2022.02.023. PMID: 35303432. Previewed by Thébaud, B. The elusive pulmonary neuroendocrine cell: How rare diseases may help solving common diseases. Dev Cell 57, 837-838, doi:10.1016/j.devcel.2022.03.016 (2022). Highlighted by Science 376, 149-150, doi:10.1126/science.abq3740 (2022).
- Su Y, Barr J, Jaquish A, Xu J, Verheyden JM, Sun X. Identification of lung innervating sensory neurons and their target specificity. Am J Physiol Lung Cell Mol Physiol. 2022 Jan 1;322(1):L50-L63. doi: 10.1152/ajplung.00376.2021. Epub 2021 Nov 10. PMID: 34755535.
- Sun X*, Perl AK, Li, R, Bell SM, Sajti E, Kalinichenko VV, Kalin TV, Misra RS, Deshmukh H, Clair G, Kyle J, Crotty-Alexander LE, Masso Silva J, Kitzmiller JA, Wikenheiser-Brokamp KA, Deutsch G, Guo M, Du Y, Morley MP, Valdez MJ, Yu HV, Jin K, Bardes EE, Zepp JA, Neithamer T, Maria C. Basil MC, Zacharias WJ, Verheyden JM, Young RE, Bandyopadhyay G, Lin S, Ansong C, Adkins J, Salomonis N, Arronow B, Xu Y, Pryhuber G, Whitsett J, Morrisey EE*, NHLBI LungMAP Consortium. A Census of the Lung: CellCards from LungMAP. Dev Cell. 2022 Jan 10;57(1):112-145.e2. doi: 10.1016/j.devcel.2021.11.007. Epub 2021 Dec 21.PMID: 34936882 *Co-corresponding authors.
- Wang A*, Chiou J, Poirion OB, Buchanan J, Valdez MJ, Verheyden JM, Hou X, Kudtarkar P, Narendra S, Newsome JM, Guo M, Faddah DA, Zhang K, Young RE, Barr J, Sajti E, Misra R, Huyck H, Rogers L, Poole C, Whitsett JA, Pryhuber G, Xu Y, Gaulton KJ*, Preissl S*, Sun X*; NHLBI LungMap Consortium. Single-cell multiomic profiling of human lungs reveals cell-type-specific and age-dynamic control of SARS-CoV2 host genes. Elife. 2020 Nov 9;9:e62522. doi: 10.7554/eLife.62522. *Co-corresponding authors.
- Li R, Li XP, Hagood J, Zhu MS and Sun, X. Myofibroblast Contraction is Essential for Generating and Regenerating the Gas-Exchange Surface. J Clin Invest. 2020 Jun 1;130(6):2859-2871. doi: 10.1172/JCI132189. PMID: 32338642; PMCID: PMC7260039
- Young RE, Jones MK, Hines EA, Li R, Luo Y, Shi W, Verheyden JV and Sun, X. Airway Smooth Muscle Differentiation is Essential for Promoting Airway Size, Tracheal Cartilage Segmentation, But Dispensable for Epithelial Branching. Dev Cell. 2020 Apr 6;53(1):73-85.e5. doi: 10.1016/j.devcel.2020.02.001. Epub 2020 Mar 5. PMID: 32142630.
- Sui P, Wiesner DL, Xu J, Zhang Y, Lee J, Van Dyken S, Lashua A, Yu C, Klein BS, Locksley RM, Deutsch G, Sun X. Pulmonary neuroendocrine cells amplify allergic asthma responses. Science . 2018 8;360(6393). pii: eaan8546.
- McCulley DJ, Wienhold MD, Hines EA, Hacker TA, Rogers A, Pewowaruk RJ, Zewdu R, Chesler NC, Selleri L, Sun X. PBX transcription factor drives pulmonary vascular adaptation to birth. Journal of Clinical Investigation , 2018, 128(2): 655-667.
- Branchfield K, Nantie L, Verheyden JM, Sui P, Wienhold MD, Sun X. Pulmonary neuroendocrine cells function as airway sensors to control lung immune response. Science. 2016 Feb, 351:707-10. Prospective of this study was published in Science , and in Nature Immunology .
Biography
Xin Sun received her Ph.D. from Yale University studying Notch signaling in Drosophila with Dr. Spyros Artavanis-Tsakonas. She carried out postdoctoral training at UCSF studying FGF signaling in mouse with Dr. Gail Martin. She was on faculty in the Laboratories of Genetics at University of Wisconsin-Madison from 2002 to 2016. She moved her lab to UCSD in 2016. Dr. Sun was the recipient of Burroughs-Wellcome career award, March of Dimes Basil O’Conner award, Romnes Faculty Fellowship by Wisconsin Alumni Research Foundation, and American Academy of Allergy, Asthma and Immunology Donald Leung lectureship. She served as Director for the Cold Spring Harbor Mouse Development, Stem Cell and Cancer course. Training students and postdocs has always been a priority and the Sun lab is a welcoming and supportive environment for scientific investigation.
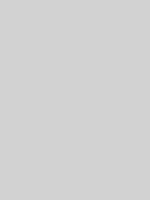