Synthetic Biology Used to Limit Bacterial Growth and Coordinate Drug Release
July 20, 2016
By Deborah Osae-Oppong
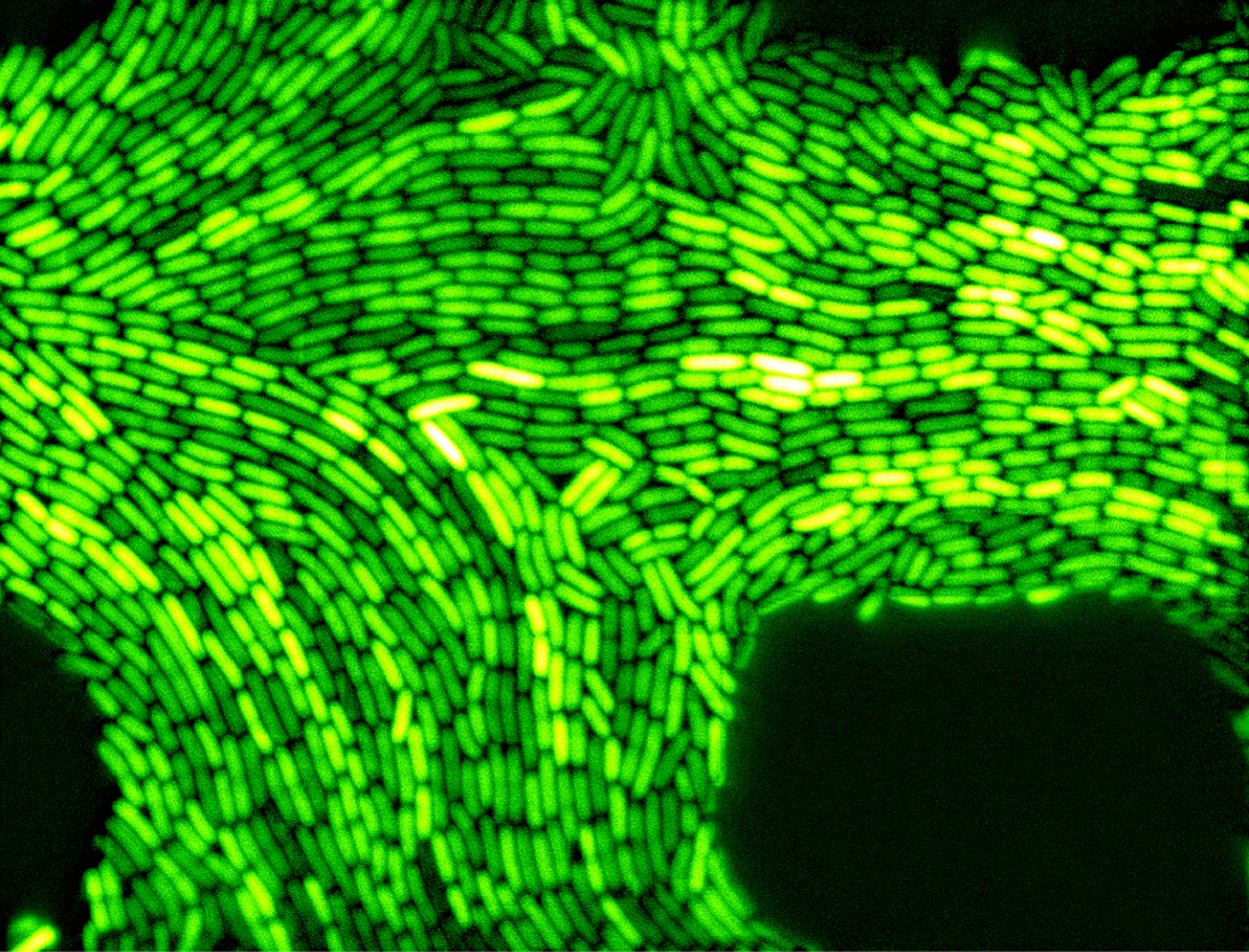
Researchers at the University of California San Diego and the Massachusetts Institute of Technology (MIT) have come up with a strategy for using synthetic biology in therapeutics. The approach enables continual production and release of drugs at disease sites in mice while simultaneously limiting the size, over time, of the populations of bacteria engineered to produce the drugs. The findings are published in the July 20 online issue of Nature.
UC San Diego researchers led by Jeff Hasty, a professor of bioengineering and biology, engineered a clinically relevant bacterium to produce cancer drugs and then self-destruct and release the drugs at the site of tumors. The team then transferred the bacterial therapy to their MIT collaborators for testing in an animal model of colorectal metastasis. The design of the therapy represents a culmination of four previous Nature papers from the UC San Diego group that describe the systematic development of engineered genetic clocks and synchronization. Over the years, the researchers have employed a broad approach that spans the scales of synthetic biology,
The new study offers a therapeutic approach that minimizes damage to surrounding cells.
In synthetic biology, one goal of therapeutics is to target disease sites and minimize damage, said UC San Diego bioengineering and biology professor Jeff Hasty. He wondered if a genetic kill circuit could be engineered to control a population of bacteria in vivo, thus minimizing their growth. We also wanted to deliver a significant therapeutic payload to the disease site.
In order to achieve this, he and his team synchronized the bacteria to release bursts of known cancer drugs when a bacterial colony self-destructs within the tumor environment. The use of bacteria to deliver cancer drugs in vivo is enticing because conventional chemotherapy doesn't always reach the inner regions of a tumor, but bacteria can colonize there. Importantly, the researchers observed that the combination of chemotherapy and the gene products produced by the bacterial circuit consistently reduced tumor size.
The new work by Jeff Hasty and team is a brilliant demonstration of how theory in synthetic biology can lead to clinically meaningful advances, said Jim Collins, a professor at MIT who is known as a founder of the field of synthetic biology. Over a decade ago during the early days of the field, Jeff developed a theoretical framework for synchronizing cellular processes across a community of cells. Now his team has shown experimentally how one can harness such effects to create a novel, clinically viable therapeutic approach.
Limiting the bacterial population
In order to observe the bacterial population dynamics, the researchers designed custom microfluidic devices for careful testing before investigations in animal disease models. Consistent with the engineering design, they observed cycling of the bacterial population that successfully limits overall growth while simultaneously enabling production and release of encoded cargo. When the bacteria were equipped with a gene that drives production of a therapeutic, the synchronized lysis of the bacterial colony was shown to kill human cancer cells. It is the first engineered gene circuit in synthetic biology to achieve these objectives.
In this paper, we describe a circuit that contains a gene that codes for a small molecule that can diffuse between cells and can turn on genes, said Omar Din, the paper's lead author and a UC San Diego Jacobs School of Engineering bioengineering Ph.D. student in Hasty's research group. Once the population grows to a critical size – a few thousand cells – there's a high-enough concentration of that molecule present in the cells to cause mass transcription of the genes behind the promoter.
The molecule, AHL, is known to coordinate gene expression across a colony of bacterial cells. Once on, the genes driven by the promoter are also activated, including the AHL-producing gene itself. Thanks to this positive feedback loop, the more AHL accumulates, the more it is produced. Because AHL is small enough to diffuse between cells and turn on the promoter in neighboring cells, the genes activated by it would also be produced in high amounts, leading to a phenomenon known as quorum sensing. Bacteria use quorum sensing to communicate with each other about the size of their population, and regulate gene expression accordingly. Scientists have used this natural ability of bacteria extensively as a tool.
Din used quorum sensing as an engineering tool to synchronize the cells and then added a kill gene that causes cells to break open (lyse) when a bacterial colony grows to a threshold. After the mass self-destruction event, a few cells remain to repopulate the colony and the resulting population dynamics are cyclical.
The lysis circuit was originally conceived for use as an aquatic biosensor, but it subsequently became clear that an exciting application could be the coordinated release of drugs when bacteria lyse in vivo, Hasty said.
Finding the right drug combination
Next, the researchers needed to find the right drug for delivery by the bacteria. They tested three different therapeutic proteins that had been shown to shrink tumors. The tests showed that the proteins were most effective when combined. They placed the genes responsible for these proteins in the circuit along with the lysis gene. They then conducted experiments with HeLa cells that showed enough protein was produced to kill cancer cells.
The testing of the therapy in mice was carried out by UC San Diego bioengineering alumnus Tal Danino while he was a postdoctoral researcher in Sangeeta Bhatia's research group at MIT. Danino is now a professor at Columbia University.
The bacteria were first injected into mice with a grafted subcutaneous tumor. This mouse model was used to visualize the bacterial population in vivo and observe their dynamics. The result was a decrease in tumor size. Danino then used a more advanced mouse model with liver metastases, where bacteria were fed to the mice. After testing a combination of the engineered bacteria and chemotherapy with this model, the researchers found that the combined therapy prolonged survival of the mice over either therapy administered alone. The researchers note that this new approach has not yet cured any mice. They did find that the therapy led to around a 50 percent increase in life expectancy, but it's difficult to anticipate how this would translate to humans. Taken together, the experiments in mice establish a proof-of-principle for using the tools of synthetic biology to engineer 'tumor-targeting' bacteria to deliver therapeutic proteins in vivo.
Developing a strategy
The new Nature paper shows the use of quorum sensing to limit bacterial population growth and release drugs. In previous Nature papers, the Hasty lab has shown how engineered cellular oscillations can be coordinated within a bacterial colony and even between thousands of interacting colonies.
This paper describes a highly innovative strategy employing synthetic biology to weaponize bacteria, said Bert Vogelstein, Director of the Ludwig Center at Johns Hopkins University and pioneer in the field of cancer genomics. The authors show that these bacteria can be used to slow the growth of tumors growing in mice. Though much further work will be required to make this therapy applicable to humans, it's just the kind of new, forward-thinking approach that we desperately need if we are to more effectively combat cancer."
Next possible steps include investigating the natural presence of bacteria in tumors and then engineering these bacteria for use in vivo and using multiple strains of bacteria to form a therapeutic community
Additionally, we are currently investigating methods for maintaining the circuit inside bacteria, said Din. Since the proteins produced by the circuit put a burden on the bacteria, the bacteria are prone to mutate these genes. Additionally, there is a selection pressure to get rid of the plasmids which harbor the genes comprising the circuit. Thus, one of our future research aims is to identify strategies for stabilizing the circuit components in bacteria and decreasing their susceptibility to mutations.
Full paper: Synchronized cycles of bacterial lysis for in vivo delivery. Authors of the paper are M. Omar Din,* Arthur Prindle, Jangir Selimkhanov, Ellixis Julio, Lev S. Tsimring and Jeff Hasty of UC San Diego; Tal Danino,* Matt Skalak, Kaitlin Allen, Eta Atolia and Sangeeta N. Bhatia of MIT.
*These authors contributed equally to this work.
This work was supported in part by the San Diego Center for Systems Biology, the National Institute of General Medical Sciences of the National Institutes of Health (GM069811), a Koch Institute Support Grant (P30-CA14051) from the National Cancer Institute (Swanson Biotechnology Center), and a Core Center Grant (P30-ES002109) from the National Institute of Environmental Health Sciences.